The DRI Catalyst used in gas-based Direct-Reduction Iron Plants. The Midrex Direct Reduction process is based upon a low pressure, moving bed shaft furnace where the reducing gas or syngas moves counter-current to the lump iron oxide ore or iron oxide pellet solids in the bed. The reducing gas is produced from the reforming of natural gas using two nickel based catalyst types and one inert ceramic support. Taking into account the very severe operational conditions in Midrex reformers due to high gas temperatures, low pressures, low steam to carbon ratio and high CO2 content in the reformer feedstock, two variants of catalyst along with an inert layer should be loaded to avoid catalyst deterioration, one with higher nickel oxide content and activity based on alumina and the other with lower nickel oxide and activity on rugged magnesium oxide carrier. Unlike conventional steam reformers, in Midrex process, feedstock moves upward inside the tubes and accordingly the catalysts loading pattern should be adjusted so that the feed gas stream is firstly pre-heated over a sufficient volume of ceramic support layer, then partially reformed and heated over a semi-active magnesium oxide based catalyst to facilitate the process conditions of the application of an alumina based high active catalyst. Hence, the ultimate reforming will be done over a high active catalyst of Direct-Reduction Iron.
Reformer Catalyst for Direct Reduction of Iron
- Inert DRI Catalyst
- Semi-active DRI Catalyst
- Active DRI Catalyst
- De-sulfurization
Several significant features of the robust DRI Catalyst Direct-Reduction Iron are as follows:
- High catalytic activity for converting feedstock
- High resistance against coke formation
- High heat transfer coefficient
- Low pressure drop and high geometric surface area
- Excellent stability of catalyst performance.
Process:
CH4 + H2O ⇌ CO + 3 H2
CH4 + CO2 ⇌ 2 CO + 2 H2
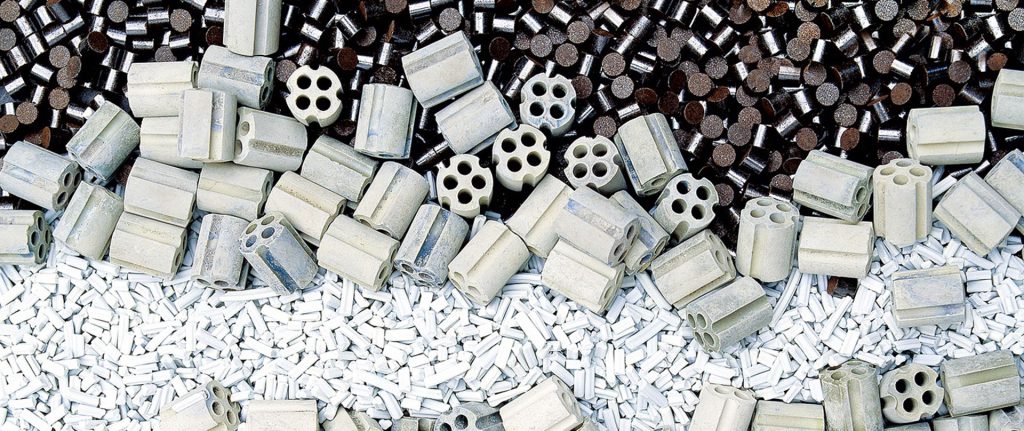
Designs of tubular steam methane reformers (SMRs) have, wherever possible, been optimized around the
limitations of key component capabilities, i.e. dimensions and high temperature creep of tubes, together with the pressure drop and activity of the conventional catalysts within the tube.
Over the years, tube and catalyst manufacturers have improved their respective products, but limitations of conventional catalyst carrier manufacturing processes have slowed any significant developments over the last 20 years.
The next generation of catalyst required a step change in carrier manufacturing processes. It is generally known that spherical shapes pack better in tubes than alternate cylindrical types, but it has been difficult to manufacture spheres in volume, so all conventional carriers have been non-spherical.